

Engineering and Technology Quarterly Reviews
ISSN 2622-9374



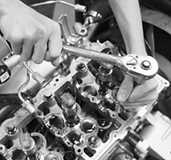



Published: 05 August 2022
Numerical Analysis of Frictional Drag Reduction of Watercraft Using Water Lubrication Technique
Atilla Uygar Sönmez, Vijayan S. N.
Gaziantep University (Turkey), Karpagam Institute of Technology (India)
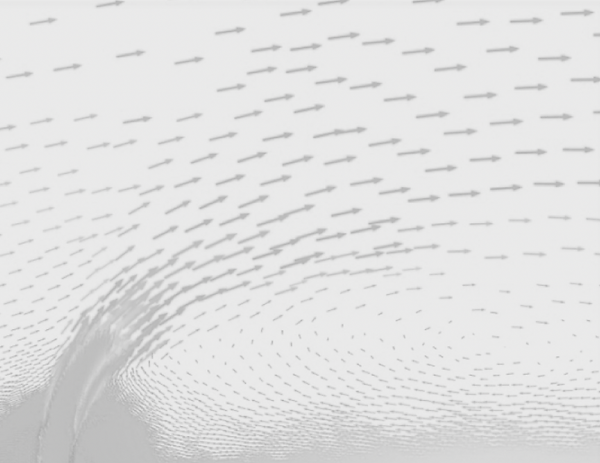
Download Full-Text Pdf

10.5281/zenodo.6965512
Pages: 18-29
Keywords: Watercraft, Friction, Drag Reduction, Water Jet, Flow, ANSYS
Abstract
The majority of the energy expended by watercraft is used to overcome drag. Frictional resistance can account for up to 80 % of overall resistance, especially on large vessels, which leads to an increase the fuel consumption and environmental impacts. As a result, finding strategies to reduce frictional drag is interesting. There are numerous strategies for reducing drag that have already been proposed that are air lubrication techniques, boundary layer energizing, and suction is few of the examples. This study proposes a new way of reducing drag this method is known as water lubrication. To create adverse flow, water jets are used to split the flow. The formation of an auspicious boundary layer ore sluggish boundary layer surrounding the surface it reduces drag significantly. Separation is generally undesired since it expands the wake zone and as a result it produces drags. In flat plate separation it does not significantly enlarge the wake region. Separations form a slow or adversely directed layer on the flat plate if they are created and positioned correctly. This is demonstrated in this study through the use of ANSYS Fluent simulation. Also, with water jet velocities of 3 to 15 m/s drag reductions of 17 % to 134 % have been achieved.
References
Munson, B. R., Okiishi, T. H., Rothmayer, A. P. (2013). Fluid Mechanics: Fundamentals of Fluid Mechanics (7thEdition). John Wiley and Sons Inc: New York.
Sturm, H., Dumstorff, G., Busche, P., Westermann, D., Walter, L., W. (2012). Boundary layer Separation and Reattachment Detection on Airfoils by Thermal Flow Sensors. Sensors. 12(11), 14292-306. https://doi.org/10.3390/s121114292.
Schlichting, H., Gersten, K., Krause, E. (2008). Grenzschicht-Theorie, 10th ed. Springer Berlin. 377–408. https://doi.org/10.1007/3-540-32985-4.
Bohl, W., Elmendorf, W. (2008). Technische Stromungslehre. 14th ed. Vogel-Fachbuch: Wurzburg, Germany, 260–302.
Uruba, V., Knob, M. (2009). Dynamics of a Boundary layer Separation. Engineering Mechanics. 16(1), 29–38.
Gad-el Hak, M. (2000). Flow Control-Passive, Active, and Reactive Flow Management, 1st ed.; Cambridge University Press.Cambridge, UK. 150–203.
David, C. H. (1968). Boundary layer Control. Film Notes. National Committee for Fluid Mechanics Films. Princeton University: Princeton.
Craven A. H. (1960). Boundary layers with Suction and Injection A review of published work on skin friction. Royal Air Force Technical College, Henlow. http://dspace.lib.cranfield.ac.uk/handle/1826/9420.
Seif, M. S., Tavakoli, M. T. (2004). New Technologies for Reducing Fuel Consumption in Marine Vehicles, XVI Symposium SORTA.
Wang, J. J., Choi, K.S., Feng, L. H. (2013). Recent Developments in DBD Plasma Flow Control, Progress in Aerospace Sciences. 62, 52-78. https://doi.org/10.1016/j.paerosci.2013.05.003.
Shimizu, K., Boundary layerajan, M. (2018). Dielectric Barrier Discharge Microplasma Actuator for Flow Control, Actuators. DOI: 10.5772/intechopen.75802.
Gerbedon, J.C.,Talbi, A.,Viard, R., Preobrazhenskya, V., Merlenb, A., & Pernod, P. (2015). Elaboration of compact synthetic micro-jets based on micro magneto-mechanical systems for aerodynamic flow control. Procedia Engineering. 120, 740 -743. https://doi.org/10.1016/j.proeng.2015.08.789.
Yanga, D., Xiong, Y. L., Guod, X. F. (2017). Drag Reduction of a Rapid Vehicle in Supercavitating Flow. International Journal of Naval Architecture and Ocean Engineering. 9(1), 35-44. https://doi.org/10.1016/j.ijnaoe.2016.07.003.
Schlichting, H. (1968). Boundary-layer Theory. 6th edition, McGraw-Hill, New York.
Vijayan, S.N., Sendhilkumar., S., Kiran Babu, K. M., Duraimurugan, G.K., & Deepak, P. (2018). CFD Analysis of Frictional Drag Reduction on the Underneath of the Ship’s Hull Using Air Lubrication System, International Journal of Mechanical Engineering and Technology (IJMET). 9(4), 408–416. https://iaeme.com/Home/article_id/IJMET_09_04_046.
David Paul, D., Vijayan, S.N., Navish Kumar. (2019). Investigation on thermal effects of Al2O3Nanoparticles mixed with water in forced convection micro channel using Computational fluid dynamics. International Journal of Engineering and Advanced Technology. 8(3),217-222. https://www.ijeat.org/wp-content/uploads/papers/v8i3/C5792028319.pdf.
Kawakita, C., Sato, S., & Okimoto, T. (2015). Application of simulation technology to Mitsubishi air lubrication system. Mitsubishi Heavy Industries Technical Review. 52(1), 50-56.
Sililberschmidt, N., Tasker, D., Pappas, T., & Johannesson, J. (2016). Silverstream system - air lubrication performance verification and design development. Conference of Shipping in Changing Climate, Newcastle, UK, 10-11.
Merryisha, S., Parvathy, R. (2019). Experimental and CFD Analysis of Surface Modifiers on Aircraft Wing: A Review. CFD Letters. 11(10), 46-56. https://akademiabaru.com/submit/index.php/cfdl/article/view/3516.
Spálenský, V., Rozehnal, D. (2017). CFD Simulation of Dimpled Sphere and its Wind Tunnel Verification, MATEC Web of Conferences. 107 (1–3), 00077.
McCormick, M. E., Bhattacharyya, R. (1973). Drag reduction of a submersible hull by electrolysis. Naval Engineering 85, 11-16. https://doi.org/10.1111/j.1559-3584.1973.tb04788.x.
YuichiMurai. (2014). Frictional Drag Reduction by Bubble Injection. Experiments in Fluids. 55 (1773). https://doi.org/10.1007/s00348-014-1773-x.
Takahashi, T., Kakugawa, A., Nagaya, S., Yanagihara, T., & Kodama, Y. (2001). Mechanisms and scale effects of skin friction reduction by microbubbles. Proc. 2nd Symp. on the Smart Control of Turbulence, University of Tokyo, 1–9.
Watanabe, O., Masuko, A., Shirose, Y. (1998). Measurements of Drag Reduction by Microbubbles using very long Ship Models. Journal of the Society of Naval Architects of Japan. 183, 53–63. https://doi.org/10.2534/jjasnaoe1968.1998.53.
Ahmed Z. Al-Garni., Abdullah M. Al-Garni., Saad A. Ahmed., & Ahmet Z. Sahin. (2000). Flow Control for an Airfoil with Leading-Edge Rotation. Journal of Aircraft.37(4), 617-622.
Shao, N., Yao, G., Zhang, C., Wang, M. (2017). A New Method to Optimize the Wake Flow of a Vehicle: The Leading Edge Rotating Cylinder. Mathematical Problems in Engineering. 1, 1-16. https://doi.org/10.1155/2017/5781038.
Diamond, P., Harvey, J., Katz, J., Nelson, D., & Steinhardt, P. (1992). Drag Reduction by Polymer Additives. The MITRE Corporation, McLean, Virginia.
Wang, J.J., Choi, K.S., Feng, L.H., Jukes, T.N., & Whalley, R.D. (2013). Recent Developments in DBD Plasma Flow Control. Progress in Aerospace Sciences. 62, 52-78. 10.1016/j.paerosci.2013.05.003.
Kazuo Shimizu., Marius Blajan. (2018). Dielectric Barrier Discharge Microplasma Actuator for Flow Control. Actuators. 10.5772/intechopen.75802.
Vijayan, S. N., Sendhilkumar, S., & Kiran Babu, K. M. (2015). Design and analysis of automotive chassis considering cross-section and material. International Journal of Current Research. 7(05), 15697-15701. https://www.journalcra.com/article/design-and-analysis-automotive-chassis-considering-cross-section-and-material.
Singarajan Nagammal Vijayan., Sathiavelu Sendhilkumar. (2015). Structural Analysis of Automotive Chassis Considering Cross-Section and Material. International Journal of Mechanical Engineering and Automation. 2(8), 370-376.
Vijayan, S.N., Makeshkumar, M. (2012). Material Specific Product Design Analysis for Conditional Failures – A Case Study. International Journal of Engineering Science and Technology. 4(03), 976-984.
Nakayama, Y. (2018). Introduction to Fluid Mechanics. 2nd Edition, Butterworth-Heinemann.
Atilla Uygar Sönmez (2022). Water Lubrication for Friction Drag Reduction. International Scientific Research Congress Dedicated to the 30th Anniversary of Baku Eurasia University, 1198-1206.