

Engineering and Technology Quarterly Reviews
ISSN 2622-9374



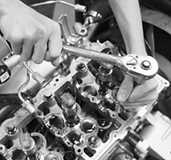



Published: 19 September 2022
A Numerical Parametric Study on the Impacts of Mass Fractions of Gas Species on PEMFC Performance
Mahmut Kaplan
Gaziantep University
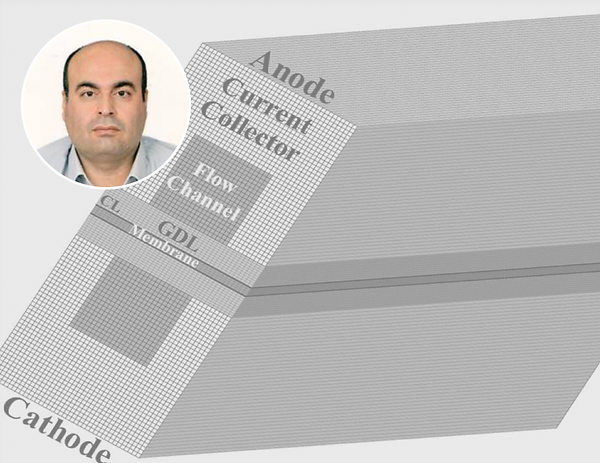
Download Full-Text Pdf

10.5281/zenodo.7089796
Pages: 38-45
Keywords: PEMFC, CFD Model, Species Mass fractions, Cell Performance, Pressure drop, Optimization
Abstract
Proton exchange membrane fuel cell (PEMFC) has attained increasing interest during the past decade owing to operating at low temperatures, generating higher efficiency power and having low pollutant emissions. Many parameters including operating temperatures, pressures and mass fraction of gas species affect PEMFC efficiency. In this work, a 3D Computational Fluid Dynamics approach is employed to study the influences of hydrogen mass fraction (0.1-0.6), oxygen mass fraction (0.1-0.2) and cathode water mass fraction (0.1-0.26) on the cell performance at 0.4-0.8 V. The numerical results using ANSYS Fluent reveal that decreasing hydrogen mass fraction and increasing water mass fraction augment the current density at 0.4 and 0.6 V. Besides, decreasing oxygen mass fraction from 0.2 to 0.16 also enhances the cell performance whereas the current density remains almost constant with further decreasing oxygen mass fraction. The highest current density of 2.30 A/cm2 is achieved with mass fraction of oxygen of 1.6 and the current density increases by 82.5% compared to the base model at 0.4 V. However, the pressure drop of channels is not significantly affected by modifying mass fractions of gases. It is found that determining the optimum value of each species mass fraction at the anode and cathode plays a crucial role in the development of the cell efficiency.
References
Ahmadi N., & Rostami S. (2019). Enhancing the performance of polymer electrolyte membrane fuel cell by optimizing the operating parameter. Journal of the Brazilian Society of Mechanical Sciences and Engineering, 41, 220. https://doi.org/10.1007/s40430-019-1720-0
ANSYS FLUENT Fuel Cell Modules Manual, Release 14.0, ANSYS Inc., November 2011.
Barbir F. (2013). PEM fuel cells: theory and practice. Elsevier/Academic Press.
Spiegel C. (2008). PEM fuel cell modeling and simulation using MATLAB. Elsevier/Academic Press.
Biyikoglu A., & Alpat C. O. (2011). Parametric study of a single cell proton exchange membrane fuel cell for a bundle of straight gas channels. Gazi University Journal of Science, 24(4), 883–899.
Kahveci E. E., & Taymaz I. (2018). Assessment of single-serpentine PEM fuel cell model developed by computational fluid dynamics. Fuel, 217, 51-58. https://doi.org/10.1016/j.fuel.2017.12.073
Kaplan M. (2021). Numerical investigation of influence of cross-sectional dimensions of flow channels on PEM fuel cell performance. Journal of Energy Systems, 5(2), 137–148. https://doi.org/10.30521/jes. 871018
Mazumder S., & Cole J. V. 2003. Rigorous 3-D mathematical modeling of PEM fuel cells II. Model predictions with liquid water transport. Journal of The Electrochemical Society, 150(11), A1510-A1517. https://doi.org/10.1149/1.1615609
Ogungbemi E., Wilberforce T., Oluwatosin I., Thompson J., & Olabi A. G. (2021). Review of operating condition, design parameters and material properties for proton exchange membrane fuel cells. International Journal of Energy Research, 45(2), 1227-1245. https://doi.org/10.1002/er.5810
Salva J. A., Iranzo A, Rosa F., Tapia E., Lopez E, & Isorna F. (2016). Optimization of a PEM fuel cell operating conditions: Obtaining the maximum performance polarization curve. International Journal of Hydrogen Energy, 41(43), 19713-19723. https://doi.org/10.1016/j.ijhydene.2016.03.136
Subin K., & Jithesh P. (2018). Experimental study on self-humidified operation in PEM fuel cells. Sustainable Energy Technologies and Assessments 27, 17-22. https://doi.org/10.1016/j.seta.2018.03. 004
Song G. H., & Meng S. (2013). Numerical modeling and simulation of PEM fuel cells: Progress and perspective. Acta Mechanica Sinica, 29(3), 318–334. https://doi.org/10.1007/s10409-013-0037-y
Xing L., Shi W., Su H., Xu Q., Das P. K., Mao B., & Scott K. (2019). Membrane electrode assemblies for PEM fuel cells: A review of functional graded design and optimization. Energy, 177, 445-464. https:// doi .org/10.1016/j.energy.2019.04.084
Wang L., Husar A., Zhou T., & Liu H. (2003). A parametric study of PEM fuel cell performances. International Journal of Hydrogen Energy, 28(11), 1263–1272. https://doi.org/10.1016/S0360-3199 (02)00284-7
Wang Y., Diaz D. F. R., Chen K. S., Wang Z., & Adroher X. C. (2020). Materials, technological status, and fundamentals of PEM fuel cells – A review. Materials Today, 3, 178-203. https://doi.org/10.1016/j. mattod.2019.06.005
Wu H. W. (2016). A review of recent development: Transport and performance modeling of PEM fuel cells. Applied Energy,165, 81-106. https://doi.org/10.1016/j.apenergy.2015.12.075
Yang Y. Jia H., Liu Z. Bai N., Zhang X., Cao T., Zhang J., Zhao P., & He X. (2022). Overall and local effects of operating parameters on water management and performance of open-cathode PEM fuel cells. Applied Energy, 315, 118978. https://doi.org/10.1016/j.apenergy.2022.118978